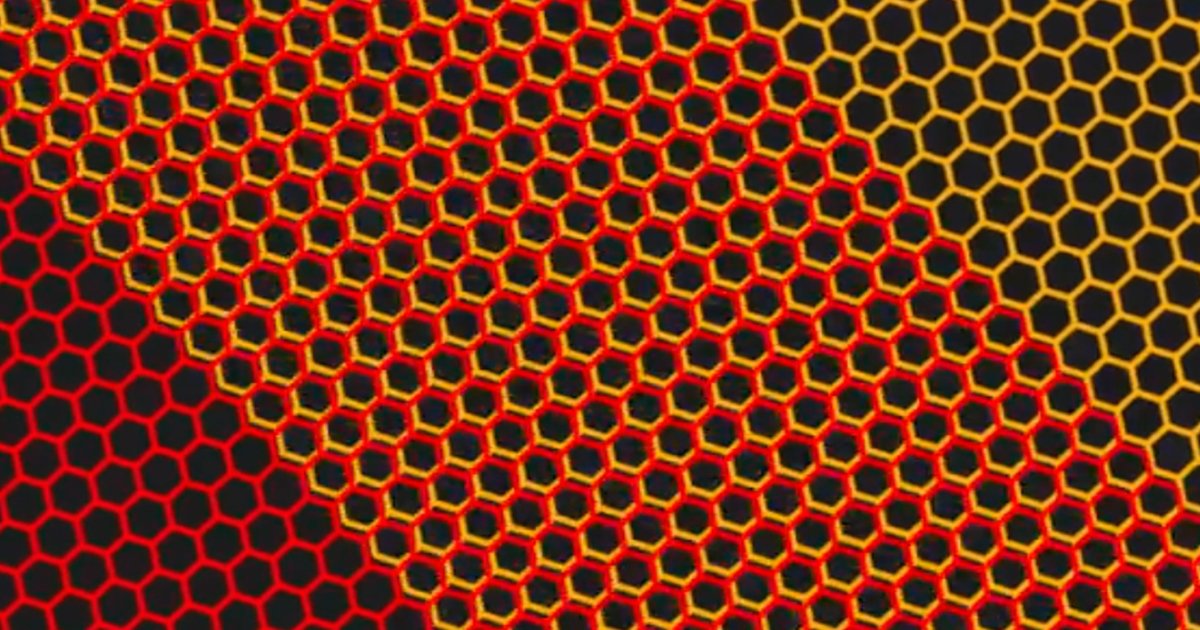
Exotic New Superconductors Delight and Confound
Theorists brainstormed and came up with new ways to pair electrons.
High-temperature superconductors appear to have atoms arranged in a way that slows down electrons. When electrons have a chance to mix in a leisurely way, they collectively create a gorgeous electric field that can make them do novel things, like form pairs instead of repelling. Physicists now suspect that in copper oxides, specifically electrons jump between atoms In a special way that favors pairing. But other “unconventional” superconductors remain quite mysterious.
Then, in 2018, a new superconductor opened the eyes of physicists.
Pablo Jarillo-HerreroMIT physicists discovered that if you take a sheet of carbon atoms arranged in a honeycomb lattice, a two-dimensional crystal called graphene, twist it exactly 1.1 degrees and stack it on top of another sheet of graphene On top of the sheet, two layers can superconduct.
Researchers have dabbled in 2D materials and discovered different behaviors. By applying an electric field, they can add electrons to the sheet, or make the electrons feel like the atomic grid is shrinking. Adjusting these settings in a single 2D rig can reproduce the behavior of thousands to millions of potential materials. Jarillo-Herrero shows that among these possibilities is a new superconductor: “magic horn“Graphene.
Then, a few years later, a team in California removed the magic angle and discovered a three-layer twist-free graphene device Can also be superconducting.
Mark Belland/Quanta Magazine
Researchers are still debating why electrons stick together in these situations. Phonons fit the data in some ways, but something new It also seems responsible.
But what really excites physicists is the promise of a new way to study superconductivity. Customizable 2D equipment frees them from the drudgery of designing, growing and testing new crystals one by one. Researchers are now able to quickly reproduce the effects of many different atomic lattices in a single device and pinpoint the capabilities of electrons.
This research strategy is now bearing fruit. This year, physicists discovered superconductivity for the first time in a two-dimensional material other than graphene, and discovered an entirely new form of superconductivity in a new graphene system. These findings suggest that early graphene superconductors were just the outskirts of a wild new jungle.
A tip turned out to be correct
2020, physicist Corey Dean and his team at Columbia University tried stacking different sheets of two-dimensional crystals—honeycomb arrangements of two types of atoms called transition metal dichalcogenides (TMDs). When they twisted the sheet 5 degrees, Resistance falls to zero But didn’t stay there. This is an uncertain hint of superconductivity.
The tentative nature of testing has not stopped Liang Fu Professor Konstantin Schrader, professor at MIT and Louisiana State University try to explain it. They suspected that phonons were not the answer. Twisted materials are powerful because the twisting changes the electron’s experience, causing the material to appear in a kaleidoscope of “moiré” patterns. Moire fringes are characterized by large hexagonal cells that act like artificial atoms, housing electrons. In this new environment, the electrons move slowly enough so that their collective electrical interactions can guide their behavior.
5W infographic; Mark Belland/Quanta Magazine
But how do electrons come together to form electron pairs? The Columbia research team concentrated electrons into moiré fringes. They observed that when each large unit in a moiré material had one electron, the electrons exhibited an “antiferromagnetic” arrangement; their intrinsic magnetic fields tended to alternate between upward and downward. Adding extra electrons to the moiré stripes reduces the resistance to zero – Cooper pairs are formed. Fu and Schrader believe that the same electron-on-electron effects make antiferromagnetic and superconducting states possible. With one electron per cell, each electron can have a preferred location and magnetic orientation. But when extra electrons pile up, the magnetic arrangement becomes unstable and the entire population begins to flow freely.
Science journals initially rejected Fu and Schrade’s paper describing these ideas because there wasn’t any conclusive evidence that TMDs could superconduct. Now there is. The Columbia research team has spent the past four years improving their ability to measure electrical resistance at low temperatures, and earlier this year they achieved a breakthrough. They assembled another two-piece device with a 5-degree twist, cooled it, and then Saw it superconducting – Observations to be published soon nature. “Lo and behold, we see the states we thought existed appearing in the right places,” Dean said. “That’s a little bit of defense.”
Fu and Schrader’s theory, confirmed by Columbia University, has now been published, but has not yet been confirmed. One way to test this is to check whether Cooper pairs can rotate as the theory predicts. This is an unusual feature because the electrons paired with the phonons do not orbit each other.
Adding electrons to antiferromagnetic metals is not the only way to achieve superconductivity in TMDs. Shortly before Columbia’s discovery, another group discovered an even more exotic form of superconductivity in the same material.
Jie Shan and Mai Jianhuione academic power couple Jarillo-Herrero, who runs a lab at Cornell University, has been searching for superconductivity in TMDs since his blockbuster discovery of twisted graphene in 2018. Try different TMD crystals, try different twist angles and temperatures, and apply electric field strengths to various materials – looking for a needle in a haystack for superconducting components.
When the needle finally emerged, it exhibited a form of superconductivity that no one had foreseen. The Columbia team started with an antiferromagnetic metal and added electrons. The Cornell team, however, started with an insulator and added nothing. Their moiré pattern (created by a milder 3.5-degree twist) slows the electrons down so quickly and interacts so strongly that they all get stuck at one electron per cell.
2024-12-09 15:47:42